Anatomy and Physiology: The Neuromuscular Junction
The Neuromuscular Junction
A myofiber is an extraordinarily sophisticated piece of cellular machinery, but, in the end, it still only does what it is told! Each muscle cell that contracts is connected to a motor neuron. Muscles, when they contract, go whole hog, which means each cell is in an all-or-nothing mode. In order for a large muscle to have a strong contraction means that every cell in that muscle must be told to contract; weaker contractions mean fewer cells contract. So how does a nerve cell tell a muscle cell to contract?
First, the nerve and muscle cells must make contact, yet the two cells don't actually touch. The junction between a neuron and a muscle fiber is called the neuromuscular junction (NMJ) (see Figure 8.3). The junction, just as in the junction between neurons, is called a chemical synapse, and there is always a space between the cells called a synaptic cleft.

Figure 8.3The parts of a neuromuscular junction. (©Michael J. Vieira Lazaroff)
The membranes of the two cells in a synapse are named because of the direction of the nerve impulse: the presynaptic membrane (the neurolemma) and the postsynaptic membrane (the sarcolemma). The message transmitted from the neuron to the myofiber is a chemical one called a neurotransmitter, and they work by changing the permeability of the postsynaptic membrane. This type of synapse, with a synaptic cleft, is a chemical synapse; heart cells, and many nerve cells, have electrical synapses, in which the cells actually touch and travel through communicating junctions called gap junctions.
Neurotransmitters and Exocytosis
Neurotransmitters are chemical messengers that travel across the synaptic cleft between neurons and neurons, or neurons and myofibers. A neurotransmitter called acetylcholine (ACh) is used at the neuromuscular junction. Acetylcholine is initially produced in the Golgi bodies (in the cell body), and then travels down the axon to the axon terminal bud in synaptic vesicles; since there is no E.R., and no Golgi bodies, in the terminal bud, the synaptic vesicles are recycled using both endocytosis, exocytosis, and mitochondria to recycle the ACh.
So what does it mean to change “the permeability of the postsynaptic membrane?” To start off, have another look again at active transport. You might remember that active transport is the movement of molecules against the concentration gradient, from a low concentration to a high concentration. In this case, using a nifty little engine called the Na+/K+ pump, sodium ions are kept at a high concentration in the synaptic cleft. The sarcolemma, when in this state, is considered polarized. Now remember, keeping up this membrane polarized takes energy, in the form of ATP. Ironically, this energy is used to keep the muscle relaxed.
At this point it is a good idea to look at what the word relaxed actually means. Most people envision relaxed as being somewhat akin to a teenager crashed on a couch, but that is nothing like relaxed muscle. Survival depends on the ability to react quickly in emergency situations, and a quick mobilization of muscles is crucial to that ability. In that sense, a relaxed muscle is a lot like a bow and arrow, with the arrow pulled back ready to be released. The bow and arrow, even though they are not moving, are primed to move quickly; just like a muscle, this state also requires energy.
The big advantage to active transport, in this situation, is that it creates a situation in which the sarcolemma can become depolarized all the more quickly. Diffusion alone is not the fastest way to move the sodium back to a state of equilibrium. The fastest way, without a doubt, is through facilitated diffusion. Facilitated diffusion requires a channel for the sodium to pass through, but if the channel were always open it would be awfully hard to maintain active transport. There has to be a way to open and close the channel when needed. When the muscle is relaxed, the channel is closed; it is only opened when the muscle cell needs to contract. It makes sense that such a channel should be kept under lock and key. The neurotransmitter is the chemical message that tells the cell to contract; the neurotransmitter, a cool molecule called acetylcholine (ACh), is the key. The ACh is kept in synaptic vesicles in the axon terminal bud. When the axon receives the message, via a similar change in the polarization of the neurilemma, the synaptic vesicle fuses with the neurilemma and releases its contents (the ACh) into the synaptic cleft through exocytosis. The key has been released; all the key needs now is a lock.
ACh, Receptors, and Facilitated Diffusion
All along the postsynaptic membrane, the sarcolemma, are receptors for the acetylcholine (ACh). The ACh binds with the ACh receptors, which open the channel, allowing the sodium ions to flood through the postsynaptic membrane via facilitated diffusion. Once again, the combination of active transport and facilitated diffusion makes this and depolarization incredibly fast (only two milliseconds!).
The only problem with this scenario is how the muscle gets relaxed again. This is easier to understand when you think about the enzyme acetylcholinesterase (ACh-esterase). When the ACh is released into the synaptic cleft, it is broken down very quickly by the acetylcholinesterase. In order to maintain a sustained contraction, the motor neuron needs to release an almost continuous supply of ACh—luckily there's a constant recycling of the neurotransmitter, or it would not be possible to contract a muscle for more than a few milliseconds!
My, Oh, Myogram!
Have you ever heard of a muscle twitch? A myogram is a graphic representation of the speed and strength of a muscle contraction (see Figure 8.4). To understand a myogram, let's start by looking at a muscle twitch. In this graph, the X-axis indicates time, and the Y-axis indicates the strength of the muscle contraction. If you look at the following figure, you will notice that a flat line indicates the relaxed muscle, and that the quick contraction and relaxation are indicated by the bell curve.
What you may not notice right away is the location of the stimulus by the motor neuron. If you look carefully you'll notice that the contraction does not start right after the stimulus, but that there is a delay called the latent period, before the muscle contraction actually starts. What is happening here, during the latent period? Think about it, for there are several steps, in order: exocytosis of the ACh, ACh binds to the ACh receptors, the channels open, and Na+ ions flood through the newly opened channels (facilitated diffusion).
The rise of the curve is called the contraction period, and the decline is called the relaxation period. The relaxation period, however, is a reversal of the latent period: The ACh is broken down by the acetylcholinesterase, the ACh receptor channels close, and active transport returns the Na+ ions to the synaptic cleft. So why is this period so much longer than the latent period? Remember, since active transport has to move the ions upstream, it does take longer, but also remember that facilitated diffusion is so much faster when it is preceded by active transport!
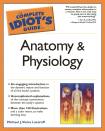
Excerpted from The Complete Idiot's Guide to Anatomy and Physiology © 2004 by Michael J. Vieira Lazaroff. All rights reserved including the right of reproduction in whole or in part in any form. Used by arrangement with Alpha Books, a member of Penguin Group (USA) Inc.
To order this book direct from the publisher, visit the Penguin USA website or call 1-800-253-6476. You can also purchase this book at Amazon.com and Barnes & Noble.